 |
Successes in Biological Control
by Anthony Shelton and Jill Eccleston
Biological control has been defined simply as the utilization of natural enemies to reduce the damage caused by noxious organisms to tolerable levels (DeBach and Rosen, 1991). One approach to biological control has been termed “classical biological control”; it involves the discovery, importation, and establishment of exotic natural enemies with the hope that they will suppress a particular organism’s population. This approach has been most successful in situations in which an organism moves or has been transported to a new environment, usually without the natural enemies that have regulated its population and prevented major outbreaks.
Sometimes such approaches have had spectacular success, as exemplified by the cottony-cushion scale project in which an introduction of a natural enemy over a century ago continues to pay dividends today. In other cases, biological control has occurred but without a documented, deliberate intervention, while in other cases continuous releases are needed to suppress pest populations.
It is also noteworthy that biological control has worked across different agricultural and landscape systems and with different types of pest species and natural enemies. There is no single “model” for whether or how biological control will work, but listed below are four examples that provide an idea of the value of biological control and hopefully provide inspiration for its future potential.

Cottony-cushion scale in California
In 1868 the cottony-cushion scale, Icerya purchasi Maskell, was discovered in the new citrus industry in California. This pest gets its common name from the white, cottony secretion it produces. The insect settles along leaf veins and sucks the phloem sap from the leaves, twigs, branches, and trunk. Feeding can result in defoliation and dieback of twigs and small branches. The impact on the California citrus industry was devastating, and no treatment was effective.
C. V. Riley, the chief of the USDA Division of Entomology, became convinced that the scale came from Australia and sent his assistant, Albert Koebele, to that continent to study the natural enemies of the I. purchasi. Koebele discovered the vedalia ladybird beetle feeding on the scale and arranged a total of 5 shipments of beetles to California from November 1888 to March 1889. A total of 514 beetles were received and released in the citrus groves of California. By 1890 all of the infestations of the cottony-cushion scale had been eliminated.
The cost of this project, aside from the salaries, was estimated to be $1,500, but the benefits to the citrus industry in California have amounted to millions of dollars annually ever since. The intriguing tale of this remarkable success is described well by DeBach and Rosen (1991) who suggest that this project “established the biological control method like a shot heard around the world”.

Control of Klamath Weed infestations on California rangeland
Hypericum perforatum Linnaeus, known also as Klamath Weed or St. John’s wort, has a long history of use as an ornamental and medicinal herb. Native to Europe, Africa, and the Middle East, this aggressive weed arrived in North America with early settlers who valued its mythical and curative powers (Mitich 1994). Unfortunately, H. perforatum also induces photosensitivity in livestock that feed upon it, leading to skin damage and related ills (Holloway and Huffaker, 1949). Wild populations of H. perforatum on California rangeland were first noted near the Klamath River around 1900. By the early to mid 1940’s, Klamath Weed had spread to over 400,000 acres of California grazing land, sickening cattle and sheep and threatening the livelihood of the state’s ranchers (Holloway and Huffaker, 1949). Controlling the weed with chemical herbicides over such a large area was considered too costly and impractical.
DeBach and Rosen (1991) described the biological control efforts against Klamath weed in California. In 1944, Professor H.S. Smith of California’s Biological Control Division received permission from the USDA to import natural enemies of H. perforatum. The war in Europe precluded collection of potential biological control agents from that continent; however, Australia, which was also fighting invasions of the weed, had already had some successes with herbivorous beetles imported earlier. Dr. A.J. Nicholson of the Commonwealth Scientific and Industrial Research Organization in Australia agreed to collect and send some of these insects to California.
A concern was that insects sourced from Australia would be unable to adjust to their life cycle to suit the reversal of seasons in the Northern Hemisphere. In Australia, the beetles spend the hot summers in a period of dormancy (aestivation), and do not become active until the arrival of winter rains. By misting the insects regularly with water, researchers were able to bring beetles from two of three imported species out of aestivation. These two species, Chrysolina hyperici and C. quadrigemina, were then put through feeding tests to ensure that they would not consume certain economically important crops (Holloway and Huffaker, 1951).
The beetles Chrysolina hyperici and C. quadrigemina were first released in 1945 and 1946, respectively. Though both species established, C. quadrigemina proved especially effective for Klamath weed control. Populations of the beetles quickly grew and spread. After 5 years, millions were collected from original release sites for redistribution throughout the Pacific Northwest. Ten years after the first releases, H. perforatum populations in California were reduced to less than 1% of their original size; “control was more effective than hoped for even by enthusiasts” (Huffaker and Kennett, 1959) and the weed no longer threatened the livestock industry. From 1953 to 1959 alone, California saved an estimated $3,500,000 per year due to this biological control program (DeBach and Rosen, 1991).

A fungus for control of gypsy moth
The tale of the gypsy moth is intriguing on several levels, including its mysterious control by a fungus (Hajek 2007). Originally brought to the US to breed with the native silkworms, the gypsy moth, Lymantria dispar L., escaped through a broken window in Medford, MA in 1868-9 and began defoliating deciduous forests and shade trees in many regions of North America. High populations of this pest are uncommon in areas where it has long been established (Europe, northern Africa and temperate Asia), but outbreaks have occurred where gypsy moth has invaded without its natural enemies. Fungal infected gypsy moths were found in Japan and brought back and released in the Boston area in 1910-11, but apparently didn’t take. By 1997, dozens of other natural enemies were also released but none provided effective control.
In the late 1980s and early 1990s, scientists noticed gypsy moth cadavers hanging from trees in the northeastern forests and identified the cause as a fungal infection. This discovery renewed interest in using fungi for control. Molecular studies indicated that the infection was caused by the same species of fungus, Entomophaga maimaiga, as the one collected in Japan in the early 1900’s, but that it was not identical to that fungus or to the same species collected and released in 1985-86. When and where did it come from and how was it spread? The answer seems that it was an accidental introduction from Japan and probably occurred after 1971. Perhaps even more interesting is that fact that it has spread across the contiguous distribution of the gypsy moth in the Northeastern US. This spread is due to aerial dispersal of spores from gypsy moth larvae and perhaps to human activity, as gypsy moths are prone to move long distances on the undersides of vehicles. There is interest in using infected gypsy moth, or the fungus itself, to inoculate the leading edges of newly infested areas and prevent outbreaks of this devastating pest. However, details of the introduction and establishment of US populations of E. maimaiga remain a mystery.

Augmentative control of greenhouse whitefly
Controlled greenhouse climates are ideal for the establishment and growth of certain pest populations. One ubiquitous invader is the greenhouse whitefly, Trialeurodes vaporariorum, a small insect with moth-like wings that feeds on a wide variety of plants. Like other homopteran insects, T. vaporariorum feeds with mouthparts that are specialized for piercing plant tissue and sucking plant juices. In addition to direct feeding injuries, whiteflies harm plants indirectly through the production of honeydew. This sticky excretion supports the growth of sooty mold, a fungal condition that blemishes plants and can interfere with photosynthesis. (Debach and Rosen, 1991).
The tiny parasitic wasp Encarsia formosa Gahan lays its eggs in immature T. vaporariorum; developing wasp larvae consume and kill their hosts before the whiteflies reach the adult stage. After E. formosa was found parasitizing T. vaporariorum on tomatoes in England in the 1920’s, a commercial industry to supply the parasitoid to greenhouses quickly developed. By the 1930’s, the wasp was commonly used for whitefly control in Europe, Canada, Australia, and New Zealand. Interest in the parasitoid waned with the advent of synthetic insecticides after World War II, but came back strongly in the 1970’s as pests became resistant to chemical treatmentsthese insecticides (van Lanteren and Woets, 1988). By the 1990’s, close to 5000 ha of greenhouse crops were employing E. formosa for control of greenhouse whitefly (van Lanteren et al., 1996).
Encarsia formosa is an example of a parasitoid that is used augmentatively, with periodic innoculative or inundative releases into greenhouses prone to whitefly populations. Wasps reared in commercial insectoriesinsectaries are distributed in the form of parasitized whitefly pupae glued to cards. Much work has been done to determine the optimal timing, spacing, and method of release of E. formosa. Hoddle et al. (1998) have reviewed some of the more common release practices, which vary depending on the host plant crop and other factors. One interesting strategy involves the use of “banker plants”, which allow the wasp to be established in a greenhouse even before a crop becomes infested with whitefly. Banker plants support a population of both T. vaporariorum and the parasitoid; fine mesh placed over these plants can prevent movement of the whiteflies, while allowing the much smaller wasp to pass freely and search for whitefly hosts on crop plants.
The greatest successes with E. formosa have been in the control of T. vaporariorum on tomato and, to a lesser extent, cucumber. Much of this success has been in Europe, especially the Netherlands and Great Britain, where there are large commercial greenhouses for vegetables and strong programs in biological control (van Lanteren and Woets, 1988).

References:
DeBach, P. and D. Rosen. 1991. Biological control by natural enemies, 2nd edition. Cambridge University Press.
Hajek, A. 2007. Introduction of a fungus into North America for control of gypsy moth. In Biological control: a global perspective (eds. C. Vincent, M. S. Goettel and G. Lazarovits). CAB International.
Hoddle, M.S., R. G. Van Driesche, and J.P. Sanderson. 1998. Biology and use of the whitefly parasitoid Encarsia formosa. Ann. Rev. Entomol. 43: 645-669.
Holloway, J. K., and Huffaker, C. B. 1951. The role of Chrysolina quadrigemina in the biological control of Klamath weed. J. Econ. Ent. 44: 244-7.
Holloway, J. K., and
Huffaker, C. B. 1949. Klamath Weed Beetles. California Agriculture. February: p. 3 and 10.
Huffaker, C. B. and C.E. Kennet. 1959. A ten-year study of vegetational changes associated with biological control of Klamath Weed. J. Range Management 12: 69-82.
Mitich, L. 1994. Common St. Johnswort. Weed Technology 8: 658-661.
van Lenteren, J. C. and J. Woets. 1988. Biological and integrated pest control in greenhouses. Ann. Rev. Entomol. 33: 239-69.
van Lanteren, J.C., H.J.W. van Roermund, and S. Sütterlin. 1996. Biological control of greenhouse whitefly (Trialeurodes vaporariorum) with the parasitoid Encarsia formosa: how does it work? Biological Control 6: 1-10.

|
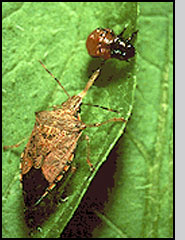 Podisus maculiventris feeding on a Colorado potato beetle larva
|
|